CLIMATE CHANGE IN URBAN AREAS F.A.Q. (MOCCIA MIX)
What is an urban heat island?
For some time it has been known that temperatures in the city are higher than those of surrounding rural areas [Meyer, 1991]. Despite receiving equal amounts of solar energy, urban centres are often observed to be significantly warmer than nearby green areas with trees and vegetation. The massive amounts of concrete that comprise the buildings and roads of an urban centre along with the vegetation that was sacrificed for them are primarily at fault. Complex city topography and generally dark colouring tends to trap sunlight during the day, and then slowly release it as infrared heat into the evening. This process drives up mean surface air temperatures, and is known as the Urban Heat Island (UHI) effect [Oke, 1982].
In more lush areas, trees offer shade and promote evapotranspiration, a process that works like air conditioning to limit the rise of air temperature [MacPherson et al., 1998]. Conversely, large cities are often jungles of concrete that don’t enjoy the benefits of a cooling breeze or shade imparted by vegetation. But asphalt, concrete and other dark building materials are only a part of the UHI story. The generation of electric power, lighting and household appliances, as well as the incessant use of automobiles greatly contribute to urban heat accumulation. Air conditioning systems are of particular concern, as the demand for air conditioning and electric power to run the devices during an urban heat wave increase quickly with temperature in a positive feedback fashion [Landsberg, HE, 1981]. To make matters worse, industrial exhaust and the burning of fossil fuels also contributes to smog that can cause further warming via a localized greenhouse effect. Unfortunately, the resulting higher temperatures accelerate the chemistry of smog formation [Heat Island Group].
UHIs have been known to exist at urban centres with only 10,000 residents and sometimes fewer [Karl et al., 1998], meaning the vast majority urbanized areas could be affected. What’s more, the United Nations Population Fund [UNPF, 1999] predicts that the majority of people will live in cities within the next two decades. UHIs have been identified world wide, and were known in London in the early 19th century even before urban warming was even understood (meteorologist Luke Howard first indicated that the city was warmer than surrounding rural areas in 1818). With urban temperatures that can be several degrees in excess of the surrounding rural areas, significant biological alterations can result. Heat stroke, dehydration and diseases can harm humans [Meyer, 1991 and references therein], and alterations in the growth and distribution of plant species have been documented [McLean, 2005]. Potential acceleration of global warming by UHIs has also been suggested, and is the topic of current research.
More importantly perhaps, methods to combat the sources of UHIs are now being developed and employed as part of a new wave of environmentally conscious building practices. Currently, alternative power technologies, urban green areas such as roof gardens, and lighter coloured more energy efficient buildings are leading the way. After all, the population is growing and much of the world is already accustomed to an overdeveloped way of life, favouring comfort over sustainability. Since urbanization cannot be stopped, the hope is that its effects can be mitigated or avoided altogether.
What causal reasons for urban heat islands?
First a definition – heat island: An urban area characterized by ambient temperatures higher than those of the surrounding [rural] area because of the absorption of solar energy by materials like asphalt [IPCC-TAR, 2001]. However, the causes leading to a UHI are many, and the actual effects of one go far beyond this definition. Figure 1 below shows the characteristically high urban temperature relative to the surrounding rural area that is rich in vegetation.
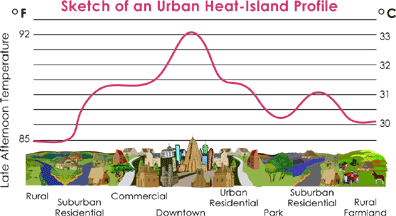
It is useful to describe the causal factors leading to the existence of a UHI so that they can be identified. First and foremost, anyone who has worn dark clothing in the summer knows that dark colours absorb heat, while white reflects it. The same is true in large, densely populated cities where asphalt, brick and concrete are the media of choice for the construction of roads and buildings, and roofs are commonly black as they are made of tarpaper, or tar and gravel. Glass itself, common to nearly every building will tend to allow solar energy inside to enter and remain trapped, leading to increased temperatures. All of these man-made materials have a low reflectivity or albedo, and function to absorb incident solar radiation [Oke, 1982]. The solar energy is then converted to heat or light in the infrared spectrum, and is reradiated throughout the day and into the night, warming the local surface air. For continental cities in the US, for example, it has been shown that the maximum heat island effect occurs a few hours after sunset [Oke, 1987] and primarily during the summer when incident solar radiation is highest [Gallo & Owen, 1999].
Conversely, plants absorb sunlight to photosynthesize and grow, and their colouring reflects infrared. Because lush vegetation rarely exists as part of the urban landscape, save a few shrubs and some grass or potted plants, there is very little protecting a city from increased temperatures. Reflectivity aside, plants also contribute to moisture in the air which produces a cooling effect, much like a cool sea breeze [Taha, 1997]. Without healthy vegetation, there is little reprieve from the summer heat waves that many communities are subjected to each summer. But recently, a NASA sponsored mission used the ATLAS sensor to study Puerto Rico as a potential UHI. Researchers found that the hottest time of the day was in the late morning (strange, since UHIs normally achieve their highest heat in the late afternoon), surpassing any cooling effects imparted by the moist sea air [Gonzalez et al., 2005].
To make matters worse, a city is often made up of tall buildings, often very close together. This topography tends to produce what is known as an urban heat canyon, whereby any solar radiation that does happen to be reflected by buildings is not sent back up, away from the surface, but is actually bounced around between the buildings and is given more chances to be absorbed or to contribute to a rise in air temperature [Chapman, 2005]. Figure 2 below illustrates this compounding effect.
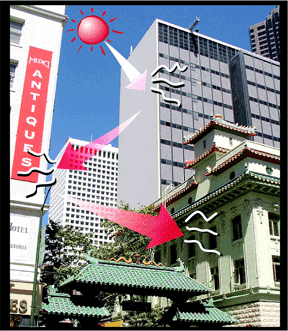
This all sounds complicated. It must be problematic to do this type of research.
It is. In fact, one overt problem associated with the study of UHIs is the difficulty in determining which urban areas can be considered heat islands. In China, for example, there are varying degrees of urbanization that are not tightly linked to population [Li et al., 2004]. In these cases, the consensus is to define a convenient population size, above which an urban area has the potential to demonstrate the heat island effect. In general this value is 10,000 residents, but heat islands have been identified with smaller populations as well [Karl et al., 1988 and Oke, 1976]. Because most cities in developed countries are much larger both geographically and in terms of population, it seems apparent that the heat island effect has the potential to affect a very large number of people.
In developed countries such as the US or much of Western Europe, researchers might determine urban areas by city lights visible from satellites at night. This type of identification is made possible through the assumption that a high proportion of the population in a well-developed metropolitan area has the same minimal access to basic necessities such as power and lighting. Clusters of light indicate urban population centres, and may be correlated with the observance of the heat island effect.
Notable research on UHIs has been conducted worldwide, extensively in China, and in the US cities Houston, Phoenix, New Jersey and Atlanta where heat waves can be deadly. As a first step, many researchers will choose sampling locations within the urban area, and among the surrounding rural area in order to record and compile temperature data from a number of locations. Currently, more advanced remote sensing methods are gaining popularity. NASA is one of the leaders in the field, with both satellite and aircraft mounted thermal/infrared sensors. The satellite data are collected by MODIS (Moderate Resolution Imaging Spectroradiometer) or ATLAS (Advanced Thermal and Land Applications Sensor), which is flown over cities using a Learjet. Other non-NASA sensors include surface thermal mapping satellites (Land Sat TM) and the NOAA DMS (National Oceanic and Atmospheric Administration Defense Meteorological Satellite) that has an AVHRR (Advanced Very High Resolution Radiometer) mounted on it. Regardless of the sensor, the two primary sensing methods involve thermal or infrared detectors and reflectivity sensors to ascertain the albedo of different materials. Some study data is summarized in the chart below, which shows the maximum difference between urban versus rural temperatures, and the method by which the data were collected.
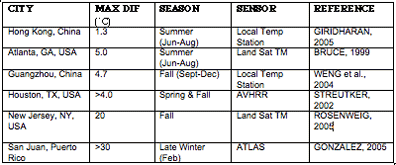
As is evident in table 1, differences in temperature can vary greatly depending on the sensor used. In general, the local temperature stations are most indicative of air temperature, which is fairly homogeneous within a given area. The aerial sensors see more rooftops and paved surfaces that are much hotter than the air above them. As well, the aerial methods cannot take into account heat that has been absorbed or reflected along the sides of urban canyons, they would only see the temperature of the lowest surface, namely the street adjacent to the base of the buildings [Voogt & Oke, 2003]. Study of UHIs using precision remote sensing equipment is becoming commonplace in climate research. The ultimate question is whether or not the increased temperatures associated with urban areas can lead to global warming.
While there are no doubts that cities warm more than forested ones, it is uncertain whether or not the UHI has led to the elevated surface temperatures noted over the last half century during rapid urbanization. The 2001 Intergovernmental Panel on Climate Change Third Assessment Report [IPCC-TAR] indicates a faster rate of warming at the surface than in the troposphere and less variation of diurnal temperatures. Both factors suggest that UHIs have an effect, in these cases localized near-surface warming, and the slow release of daytime heat into the evening long after the sun has set. The magnitude of the warming due to the UHI effect is measurable, with most study data indicating mean surface warming to be under 0.1˚C if not less than 0.05˚C over the past half century. These values are in agreement with the findings of the IPCC, and seem to account for recently observed global warming at least in some capacity.
However, some researchers find no significant contribution of UHIs to increased global temperature. They argue that when data are corrected for elevation, latitude, time of observation, and instrumentation, the presence of urban heat islands has no effect on long-term temperature rise [Peterson TC, 2003]. Another study [Li et al., 2004] compared background warming with the warming imparted by the UHI effect and found no significant contribution to long term warming. More investigation will be required to determine weather or not large differences in urban versus rural temperatures will accelerate widespread warming scenarios.
So what can be done about UHIs?
In order to protect ourselves from increased risk of heat related health hazards such as heat stroke, dehydration, and declining air quality due to smog; it is necessary to research ways to combat those factors that lead to a UHI. Essentially, any method used to reintroduce greenery into areas that were previously tar, asphalt, or concrete will help minimize UHI temperature increase. Already in use are roof gardens that absorb sunlight but reradiate very little heat. As well, the additional moisture imparted by these gardens will yield an evapotranspiration cooling effect. The more elaborate roof gardens may even include large trees if the soil depth allows. Currently there is a push to develop soils that combat excess runoff and prevent nutrients from being leached out with precipitation. One company called ERTH has developed the HydRocks soil mix that does just this [Sherman, 2005]. Increasing the amount of parkland may also prove effective at combating the UHI effect, but may be more difficult in a dense city whose overhead view is dominated by rooftops and roadways.
As a minimum measure, researchers have begun to consider the effects of using lightly coloured roofing materials. If a roof garden is not possible, simply changing the colour of a city’s rooftops could do wonders for reflecting incident solar radiation. Better building insulation and tinted or reflective windows would also help to improve a city’s albedo, lessening the effects of a pronounced summer heat wave. Improved roofing materials could function to keep the interior of a building cooler, reducing the dependence on air conditioning, which is a highly power dependent technology [Parker et al., 1994]. The use of air conditioning places heavy demands on energy production that in turn leads to increased greenhouse gas emissions, especially in areas where hydroelectric power systems are not available. The US department of energy has estimated that the use of air conditioning accounts for 1/6 of total power consumption, at an annual cost of over 40 billion dollars [Rosenfeld et al., 1997].
The persons at risk for detrimental health effects related to the UHI effect are most often the poor that cannot afford housing with air conditioning or good air circulation, the young or very old or otherwise health-impaired, and those that live on upper floors of buildings where heat trapped by the roof translates to the warmest temperatures in the building [Rozenweig, 2005].
The greenery aspect sounds interesting. Can you tell me more?
Greenery can combat the UHI effect in two main ways. Firstly trees and vegetation provide shading, preventing sunlight from reaching concrete surfaces that are susceptible to warming. Already in use are roof gardens that utilize sunlight for growth and reradiate very little heat. As well, the additional moisture imparted by these gardens will yield an evapotranspiration cooling effect, using solar energy to evaporate water rather than warm the surroundings [Heat Island Group]. In this way, vegetation functions as natural air conditioning for the same reasons that skin feels cold when it is wet. Increasing the amount of parkland is effective at combating the UHI effect, but may be more difficult in a dense city whose overhead view is dominated by rooftops and roadways. For this reason, rooftop gardens are of high value, because they help cities remain at cooler temperatures without hindering development. Where parklands require costly land within a city to exist, roof gardens could be developed along with the building upon which they would grow.

Regardless of its form, added greenery has beneficial cooling and aesthetic effects, but the more elaborate roof gardens may even include large trees if the soil depth allows. Large trees have the added bonus of being able to sequester more carbon than small ones [Washington Advisory Group LLC, 2002]. Additionally, the presence of large trees can be used more effectively to shade sunlight on the south and west sides of a building, thus preventing warming of the building later in the day when the UHI effect is most pronounced [Pomerantz et al., 1999]. Considering the type of trees planted is also important, with deciduous trees proving most effective because they shade sunlight in the summer, but lose their leaves in the winter allowing the building to warm. The main benefits come from added shade in the summer to alleviate cooling costs, and would offset the added heating costs in the winter if the shading were to persist year round. The reason why roof gardens allow for the greatest savings in the summer is because at that time the sun has a high azimuth, shining directly down on a building, where in winter the sun would shine in at an angle, focused more on the sides of a building. Thus, a roof garden may not hinder winter solar heating to an appreciable extent. In fact, trees planted around a building reduce wind speeds, thus retaining more heat in the absence of wind chill [Davis et al., 1992].
Less obvious benefits include the preservation of local species that would normally lose living space in a highly urbanized environment. With added green spaces these species need not be driven away, and thus could reside in a large city with less disruptive pressure. In addition, vegetation is known to freshen the air by filtering out pollutants such as ozone, sulfur dioxide, nitrogen dioxide, particulate matter, and carbon monoxide, alleviating the excess smog and potential for acid rain known in many cities today [Solecki et al., 2005 and Taha, 1996]. Soils that retain rainwater for use by vegetation would also limit urban runoff, reducing the tar and other chemicals that normally drain off a conventional roof [Heat Island Group].
Effective research and planning is necessary to make green roofs the industry standard in densely populated cities. Of primary concern is the strength of the roof to support the soil and trees or shrubs that will be planted. With proper planning, a harmony between aesthetically enhanced green leisure space and effective UHI mitigation can be reached. Also a matter of importance is that the soils used on a rooftop are necessarily different than the average topsoils used in conventional gardens. Currently there is a push to develop soils that combat excess runoff and prevent nutrients from being leached out with precipitation. One company called ERTH has developed the HydRocks soil mix that does just this [Sherman, 2005]. Other considerations when designing a green roof include choosing a proper sealing membrane below the soil layer to prevent moisture ingress into the building. However, the presence of vegetative root systems could actually inhibit leaks by preventing standing water.
So far, many cities have already begun to enhance green space and install roof gardens into both new and existing buildings. In Chicago, city hall has been retrofitted to include a shade providing roof garden [Brake, 2001] and in southern US cities, the energy savings from green roof upgrades are already beginning to be realized. In New York, regional climate models indicate an average cooling of 2˚C due to the efforts of resurfacing and enhancing greenery [Taha et al., 1999] Energy savings will differ depending on local weather conditions, but buildings in Florida are reporting savings of $35 to $140 USD [Parker, et al., 1994]. With an average planting cost of approximately $45 USD per tree, it is certainly not prohibitive to integrate a roof garden into any building design, and the long term benefits are well worth it. [Rosenfeld et al., 1998].
So what’s the take home message?
While we know that cities are susceptible to increased temperatures due to the low albedo of asphalt, tar, and concrete, it is not known whether or not this trend will contribute to a long-term temperature rise. So far, research indicates only mild overall warming due to the UHI effect, even though localized differences between urban and rural stations can be up to 30˚C. The development of increasingly high resolution imaging devices for use in remote sensing studies of potential UHIs, a greater knowledge of the phenomenon will no doubt become clearer with time.
What is clear, however, is that human casualties due to heat stress and air pollution are occurring due to summer heat waves in cities classified as UHIs. For those that can afford air conditioning, these effects may not be devastating, but the less fortunate urban residents can be gravely harmed.
The planting of trees and reintroduction of parkland into dense urban areas will ameliorate the occurrence of summer heat waves, and will prevent personal health risks for compromised residents. These improvements could be done at a reasonable cost, with significant environmental benefits including carbon sequestration and improved air quality. Where more reflective materials are being employed for all types of urban surfaces, roof gardens are proving effective as well, as they are functional and aesthetically pleasing. Trees planted strategically will not only reduce the demand on air conditioning during the hot summer, but will also preserve warmth in the winter by blocking cold winds. Deciduous trees that lose their leaves are optimal since they provide shade in the summer but allow solar warming in the winter when they have lost their leaves. The trees would also preserve animal habitat, keeping the urban environment more connected with nature.
Researchers with expertise in biology, climatology, engineering, remote sensing and materials science continue to elucidate and find solutions to the urban climate observations that are due to the urban heat island effect. With effective planning, cities could maintain cooler temperatures, to the benefit of all residents and local wildlife. For now, the idea of the urban heat island is gaining widespread acknowledgement, and mitigative techniques are being put into practice.
References
Brake A.G., 2001. Rooftop Oasis. Architecture vol. 90, no. 6, pp. 54-55.
Bruce, V., 1999. Atlanta’s urban heat island. NASA Goddard Space Flight Center Scientific
Visualization.
Chapman, David M., 2005. It’s HOT in the City! Geo Date, vol. 18, no. 2, pp. 1-4.
Davis S. et al., 1992. Planting and light coloured surfacing for energy conservation in cooling our communities: a guidebook on tree planting and light coloured surfacing. EPA guidebook 1992, pp. 91-110.
Gallo, K. P., and T. W. Owen, 1999: Satellite-based adjustments for the urban heat island temperature bias. Journal of Applied Meteorology, 38: 806–813.
Giridharan R., et al., 2005. Nocturnal heat island effect in urban residential developments of Hong Kong. Energy and Buildings 37: 964–971.
Gonzalez, J.E., et al., 2005. Urban heat islands developing in coastal tropical cities. EOS, Vol. 86, No. 42, pp. 397, 403.
Intergovernmental Panel on Climate Change Third Assessment Report (IPCC-TAR), 2001. Climate Change 2001: The Scientific Basis. Contribution of Working Group I Houghton, J.T. et al. (eds.) Cambridge University Press, 881 pp.
Karl, T.R., et al., 1988. Urbanization: its detection and effect in the United States climate record. Journal of Climate 1: 1099–1123.
Landsberg, H.E., 1981. The Urban Climate. National Academy Press, New York 275pp.
Li Q., et al., 2004. Urban heat island effect on annual mean temperature during the last 50 years in China. Theoretical Applied Climatology 79: 165–174
MacLean MA, et al., 2005. If you can’t stay out of the heat, stay out of the city: Thermal reaction norms of chitinolytic fungi in an urban heat island. Journal of Theoretical Biology 30: 384-391
MacPherson, E.G. et al., 1998. Estimating cost effectiveness of residential yard trees for improving air quality in Sacramento, California, using existing models. Atmospheric Environment 32: 75–84.
Meyer W., 1991. Urban heat island and urban health: early American perspectives. Professional Geographer, 43(1), 1991, pp. 38-48
Oke TR., 1987. Boundary Layer Climates. 2d ed. Routledge, 435 pp.
Oke, T.R., 1982. The energetic basis of urban heat island. Journal of the Royal Meteorological Society 108: (455), 1–24.
Oke, T. R., 1976: City size and the urban heat island. Atmosphere.Environment, 7: 769–779.
Parker D. & Barkaszi S. 1994. Reflective roofs can reduce cooling costs. Consumers’ Research Magazine; Jul94, Vol. 77 Issue 7, p18-21.
Pomerantz M. et al., 1999. Reflective Surfaces for cooler buildings and cities. Philosophical Magazine B Vol. 79 No. 9, 1457-1476.
Rosenfeld A.H. et al., 1998. Cool communities: strategies for heat island mitigation and smog reduction. Energy and Buildings 28: 51-62.
Rozenwieg, C., et al., 2005. Characterizing the urban heat island in current and future climates in New Jersey. Environmental Hazards 6: 51–62.
Shahgedanova, M. & Burt, T., 1998. Urban heat islands. Geography Review, vol. 11, no. 3, pp. 36-41.
Sherman, R., 2005. Compost plays a key role in green roof mixes. Biocycle Journal of Composting and Recycling.
Solecki W.D. et al., 2003. Mitigation of the heat island effect in urban New Jersey. Environmental Hazards 6: 39-49.
Streutker D.R., 2002. A remote sensing study of the urban heat island of Houston, Texas. International Journal of Remote Sensing, Vol. 23, no. 13, 2595–2608
Taha H., 1999. Impacts of large-scale surface modifications on meteorological conditions and energy use: a 10-region modeling study. Theoretical and Applied Climatology 62: 175-185.
Taha, H., 1997. Urban climates and heat islands: albedo, evapotranspiration, and anthropogenic heat. Energy and Buildings 25: 99–103.
Taha H., 1996. Modeling impacts of increased urban vegetation on ozone air quality in the south coast air basin. Atmospheric Environment Vol. 30 no. 20 pp. 3423-3430.
United Nations Population Fund, 1999. The state of world population 1999. 76 pp. New York.
Urban Heat Island Group. Last updated August 30, 2000.
Voogt, J.A., & Oke, T.R., 2003. Thermal remote sensing of urban climates. Remote Sensing of the Environment 86: 370-384.
Washington Advisory Group LLC, May 2002. Sequestering carbon emissions in the terrestrial biosphere.
Weng, Q. & Yang, S., 2004. Managing the adverse thermal effects of urban development in a densely populated Chinese city. Journal of Environmental Management 70: 145-156.
(Images by Jane Wang)